Sunday, March 25, 2007
Thursday, March 22, 2007
Stud Welding
Typical Uses Of The Arc Stud Welding Process
- Major design specifications may be readily adapted to the process, such as material types, metallurgical aspects,s pecial thread designs, fit-up tolerances, etc.
- Capable of producing a full cross section weldment with a minimum of base metal distortion or surface disruption.
- Can be used in locations which do not permit use of other fastening methods.
- Process lends itself to the automated concept or high production shops.
- Capable of producing welds which are less costly per unit than other methods.
- Process may be effectively utilized with a minimum of time spent in personnel training.
- Process may be effectively used in maintaining weld quality with a minimum inspection time.
Posted by
Ardne
at
8:38 PM
0
comments
Labels: Stud Welding
Wednesday, March 21, 2007
Titanium Welding
Welding titanium is widely used in many application, example popellant motor case, high pressure storage vessel, jet engine components and steam turbine condensor. Being a reactive metal will react with oxygen, nitrogen, hydrogen, carbon and most refractories and metal.
In general, welding of titanium and its alloys can be readily performed, but it is necessary to exclude reactive gases, including oxygen and nitrogen from the air, and to maintain cleanliness. Thus weld properties are heavily influenced by welding procedures, especially by the adequacy of inert gas shielding.
The GTAW (gas tungsten arc welding) process is common, although GMAW (gas metal arc welding), friction welding, laser welding, resistance welding, plasma arc welding, electron beam welding, and diffusion bonding are all used in some cases. Both alloy composition and microstructure are important in determining weldability, with the presence of beta phase having a deleterious effect.
Unalloyed titanium and alpha alloys are generally weldable and welded joints generally have acceptable strength and ductility. Postweld stress-relief annealing of weldments is recommended. Some alpha-beta alloys, specifically Ti-6Al-4V, are weldable in the annealed condition as well as in the solution treated and partially aged condition (aging can be completed during the post-weld heat treatment). Strongly stabilized alpha-beta alloys can be embrittled by welding, the result of phase transformations occurring in the weld metal or the heat affected zone. Some beta alloys are weldable in the annealed or the solution treated condition.
Welding Environment Most titanium welding today is done in the open fabrication shop, although chamber welding is still practiced on a limited basis. Field welding is common. Wherever the welding is done, a clean environment is necessary in which to weld titanium. A separate area, specifically set aside for the welding of titanium, aids in making quality welds. This area should be kept clean and should be isolated from dirt-producing operations such as grinding, torch cutting and painting. In addition, the welding area should be free of air drafts and humidity should be controlled.
Welding Processes
Titanium and its alloys are most often welded with the gas tungsten-arc (GTA or TIG) and gas metal-arc (GMA or MIG) welding processes. Resistance, plasma arc, electron beam and friction welding are also used on titanium to a limited extent. All of these processes offer advantages for specific situations. However, the following discussion will be concerned primarily with GTA and GMA welding. Many of the principles discussed are applicable to all processes.
Gas Tungsten-Arc (GTA) and Gas Metal-Arc (GMA) Welding
The GTA process can be used to make butt joints without filler metal in titanium base sheet of up to about 1/8-inch thickness. Heavier sections generally require the use of filler metal and grooved joints. Either the GTA or GMA welding process can be used, although GMA welding is more economical for sections heavier than about one-half inch. If the GTA process is used, care should be exercised to prevent contact of the tungsten electrode with the molten puddle, thereby preventing tungsten pickup.
Posted by
Ardne
at
10:01 PM
0
comments
Labels: Titanium Welding
Friday, March 9, 2007
TIME- and TimeTwin welding
Last but not least, new materials and applications have opened up new performance standards and a wide range of applications for the MIG/MAG welding process, as has the increasing use of technically mature microelectronics and digital technology. Jobs with particular welding power requirements however call for a specialised variant of MIG/MAG welding: “TIME” welding. For very long weld seams with a large cross-section and a high deposition rate, the TIME process is currently the most economical solution.
Basic system principle
In contrast to conventional MIG/MAG welding, the TIME process has a longer, free wire tip in the welding torch (referred to as greater “stick-out”), as well as a faster wire speed and modified shielding gases. By definition, high-performance welding processes have one or more solid wires with a 1.0 mm or 1.2 mm diameter, with a wire feed speed of more than 15 m/min. Processes with a greater wire diameter or with flux-cored wires also count among the high-performance welding processes if the deposition rate is more than 8 kg/h.
The TIME high-performance process can be carried out as manual, mechanised or automated. Efficient power source technology contributes to successful application of this process, as do electrodes with good conveying properties and shielding gases tailored to the particular case. The most powerful TIME variant has two wires that melt simultaneously in one weld pool. In this “TimeTwin” process, the contact tubes in the special welding torch are insulated from each other, so that the material transfer for each filler wire is selectively controlled. The result is doubled or tripled welding speed compared to MIG/MAG welding, with the best possible quality and virtually no welding spatter.
Shielding gases used include inert gases with an active gas component. This normally means a mixture of gases, the main component being argon, and also incorporating helium, carbon dioxide and oxygen. Tests have shown that for many applications, excluding one of these components brings optimum results. However, argon is always the main component. In many cases, helium contributes significantly to speeding up welding. The reason for this is the very hot TIME arc on the one hand, and the high heat conductivity of helium on the other, which also contributes to optimum joining of rising edges of the weld seam. The right amounts of carbon dioxide and oxygen to use also depend on the application.
Machine technology
In principle, the power source technology corresponds to the MIG/MAG application, as do the wire feed and welding torches. In design and performance, the components are specifically adapted to the requirements of TIME high-performance welding. Therefore, time manual welding torches already have an adjustable contact tube for stick-out control and a dual circuit cooling system. The latter cools the gas nozzle directly and at full power also ensures a high duty cycle, as well as making detachment of welding spatter easier.
A special feature of the TimeTwin welding process is the separate welding potential, each of the two filler wires with its own power source. A synchronisation unit on the power sources ensures that material transfer on the filler wires occurs at the same time. Wire movement and welding current curves are separate for each arc.
Application and advantages
TIME and TimeTwin high-performance welding is primarily applied in machine construction, steel engineering, crane construction, shipbuilding, vehicle manufacture and boiler manufacturing. Materials in those applications include unalloyed steels, low-alloy steels, unalloyed structural steels, fine-grained structural steels and steels resistant to low temperatures. The process offers a whole range of benefits. The deposition rate is more than 10 kg/h. The helium component of the shielding gas produces a deep fusion penetration and provides dynamic, very strong weld seams. Indicators of good weld properties are the clean seam surfaces, flush weld toes, and the marked decrease in welding spatter. There is no need for any rework. The suitability of high-performance welding processes for all sheet thicknesses is proof of the high degree of flexibility they offer. Even difficult welding positions do not require a reduced deposition rate, and the moderate heat application keeps component distortion to a minimum.
In contrast to TIME single-wire welding, TimeTwin welding is restricted to mechanised and automated applications due to its two filler wires. Particularly with pulsed arcs, droplet detachment from the two filler wires occurs without any reciprocal influence allowing the welding of even aluminium alloys. The benefit of the two-wire process as opposed to the single-wire process is in improved control of the weld pool. This allows a substantially higher energy input, resulting in an increase in the welding speed. Equally beneficial is the delayed activity of the second arc, producing the effect of increased outgassing TIME and therefore significantly reduced pore formation.
Summary
TIMEand TimeTwin high-performance welding are exceptionally well-suited to the increased welding power and burn-off rate. Increased flexibility means that the user can weld reproducible, low-spatter weld seams at very high welding speeds. The high-performance welding processes is suitable for use mainly in steel-processing sectors with large, welding-intensive components.
Fronius Inc.
Posted by
Ardne
at
12:48 PM
0
comments
Labels: Twin Wire
Thursday, March 8, 2007
Defect In Welding
This subsection does not provide a complete overview of possible welding defects but limits itself
to types of defects that can be prevented by relatively simple measures. In addition to a description of the defects and its causes, the following tables also contain possible countermeasures that may have a favourable effect. Refer to standards, welding guidelines and other literature for more detailed information.
Occurrence and Prevention of Defects
The following list provides explanations for possible unfavourable phenomena when welding steels and presents measures to prevent these defects. Basically speaking most of the phenomena listed can be reduced by optimising the chemical composition of steel and filler metal. The list is not ranked according to the importance of the defects described.
1. HYDROGEN-INDUCED CRACKS
COUNTERMEASURE
- Use filler metals that lead to a very low hydrogen content in the weld metal.
- Re-dry the filler metal.
- Prevent the application of high stress.
- Preheat the joint.
- Use low-hydrogen postweld annealing.
- Reduce hardness increases in the weld
- metal and the heat-affected zone.
Generally speaking the ductility of specific parts of the heat-affected zone compared with the base metal is adversely affected by coarse grain formation or hardness increase.
COUNTERMEASURE
Select the optimum welding temperature cycle.
3. SOLIDIFICATION CRACKS
These may be precipitated in the centre of the bead during solidification. They are the result of
the formation of low fusion films around the grain boundaries. These films reduce the deformability of the weld metal and longitudinal cracks may form due to shrinkage stresses on solidification of the weld metal.
COUNTERMEASURE
- Modify the welding parameters so that the individual
- beads become broader and flatter, i.e.
- reduce the depth to width ratio of a bead.
- Reduce dilution with the base metal.
- Reduce the welding speed.
- Note: solidification cracks rarely occur in steels
- with low proportions of sulphur and phosphorous.
Posted by
Ardne
at
11:07 AM
0
comments
Labels: Welding Defect
Wednesday, March 7, 2007
Automatic Multiwire GMAW Multiplies Productivity
Doubling welding wires can more than double production line throughput
Originally popular in submerged arc welding, tandem-wire technologies were extended to the automated gas metal arc welding (GMAW) process in the early nineties.1 Since their introduction, more than 1000 tandem- wire GMAW systems have been installed worldwide, most replacing single-wire systems that had been pushed to the limits of their weld metal deposit rate and productivity range - Fig. 1.
Fig.1 - Tandem-wire GMAW deposition rates compared to single-wire processes.
The tandem-wire GMAW process employs two electrically isolated wire electrodes, one behind the other in the direction of welding - Fig. 2. The first wire electrode is referred to as the "lead electrode" and the second as the "trail electrode." The spacing between the two wires is close enough to enable them both to deliver metal to a single weld pool. The lead electrode generates most of the base metal root penetration, while the trail wire controls the weld pool for bead contour and edge wetting while it adds to the overall deposition rate.

The process works best with a large- diameter lead wire and a small-diameter trail wire. The larger lead wire may represent as much as 65% of the total process deposition rate while providing greater process penetration. Focused on the back edge of the molten weld pool, the trail wire, drawing a lower current, is better able to cool and control the pool. Sometimes wires of equal diameter are specified because of inventory constraints or if the welding direction is reversed somewhere on the weldment, but this compromise limits travel speed and reduces the productivity of the process - Fig. 3.

Tandem-wire GMAW depends on specialized power control software to manage the stable operation of two independent direct current welding arcs working in very close proximity, where disruptive electromagnetic influences would cause severe instability if not precisely regulated.
Fig.4 - Typical configuration of a hard-automated tandem-wire GMAW system.

Equipment ConfigurationIn order to provide individual parameter control for each of two separate, electrically isolated welding arcs, a pairing of equipment is required: two specially designed inverter power sources, two wire drives, and two separate welding wire payoff sources - Fig. 4. The power sources rely on fast digital control and specialized software. Welding parameters are set at the power sources via digital communication from a programmable logic controller associated with a dedicated hard-automation workcell or by a robot controller.

The tandem-wire welding gun (Fig. 5) is a critical component of the system, engineered with specific contact tip alignment and spacing to achieve proper arc control. To withstand high-amperage, high-duty-cycle production runs, welding guns are generally rated at the total current flowing in both electrode wires - typically in the 600- to 1200-A range. The maximum current specified for each wire is typically in the 400- to 800-A range.
Process BenefitsThe tandem-wire GMAW process can dramatically increase travel speed in sheet metal welding, and significantly increase weld metal deposition rates in heavy plate welding. The increased productivity of the tandem-wire GMAW process can improve the profitability of existing automation. In addition, it may justify the cost of new automation equipment since it can reduce the number of weld stations that would otherwise be needed and shorten payback periods associated with investment in welding automation.
Fig.6 - Root filling capabilities on 0.100-in. material at 100 in./min.
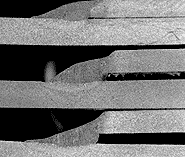
Welding operators involved in thin-gauge metal industries such as automotive, tank, and general sheet fabrication, are traditionally faced with two quality issues - melt-through and lack of metal follow characteristics - when pushing travel speeds to the limit.

Operating at speeds in excess of 100 in./min (with 0.040- to 0.120-in. materials), the tandem-wire GMAW process addresses both of these speed-limiting issues. Distributing the welding current over two wires allows the lead wire to generate the required joint penetration while the trail wire rides on the back edge of the lead weld pool, creating added fill as well as additional force against the weld pool for better follow and wetting characteristics. This riding of the weld pool with the trail arc provides excellent root filling capabilities, especially important to industries processing large numbers of stamped or formed parts - Figs. 6, 7.
Fig.8 - Eight-ft truck bolster plates being joined with tandem-wire GMAW. A 5Ž16-in. fillet weld

In heavy plate fabrication, the tandem-wire GMAW process can represent a 30 to 80% increase in deposition potential compared to single-wire processes - Fig. 1. The tandem-wire process typically employs smaller-diameter (0.035 to 0.062 in.) electrodes. As higher welding currents are applied, the electrode melt-off rate rises geometrically. For a given current draw, the melt-off rate for the tandem-wire process is greater than that of a single wire of larger diameter. This higher melt-off rate leads to higher production throughput, while the lower amperage draw (and lower heat input) reduces plate distortion and cooling time between multipass welds. The process is capable of producing X-ray quality welds with excellent mechanical properties - Fig. 8.
Return on Investment The tandem-wire GMAW process was designed for use in automated welding cells or automated lines. Investment in these high-volume production lines is a capital expenditure that must be cost-justified. Welding-speed-critical floor-to-floor time of parts is an important factor in the equation. Compared to single-wire processes, tandem-wire GMAW's higher travel speed capabilities can help justify greater capital expenditures and accelerate equipment payback periods - Figs. 9, 10.

Tandem-wire GMAW systems can reduce the cost of new production lines by meeting output needs with fewer welding stations. This is particularly true for high-volume production lines producing automotive components or similar parts where tooling and part-handling equipment constitutes a sizeable portion of the initial installation cost. Using fewer welding stations with a higher per-station throughput may reduce the cost of expensive tooling and handling equipment. Additionally, the expenses of upkeep and maintenance time for managing consistent part dimensions coming off of duplicate tooling sets are minimized.
Fig.10 - Production capabilities of single-wire vs. dual-wire GMAW.

Cost-justification for workcells welding large components (for example, earth-moving equipment or offshore drilling rigs) is based more on welding time than part count. These cells utilize capital-intensive positioners to handle large, heavy weldments that can take two or more hours to weld, often in a flat or horizontal position that allows access for only one robot per cell. In order to improve the return on these large cells, overall welding time must be reduced by increasing the weld metal deposition rate. The industry has responded by replacing single-wire robotic systems, with average deposition rates of just 1520 lb/h, with tandem-wire systems operating in the 2834 lb/h range. The increased weld metal deposition rate has been used to justify the cost of purchasing new, more technically sophisticated workstations.
Article by :
TIM MOREHEAD is Product Manager Tandem MIG Process, at The Lincoln Electric Company, Cleveland, Ohio, (216) 481-8100.
Posted by
Ardne
at
1:21 PM
0
comments
Labels: Tandem Welding